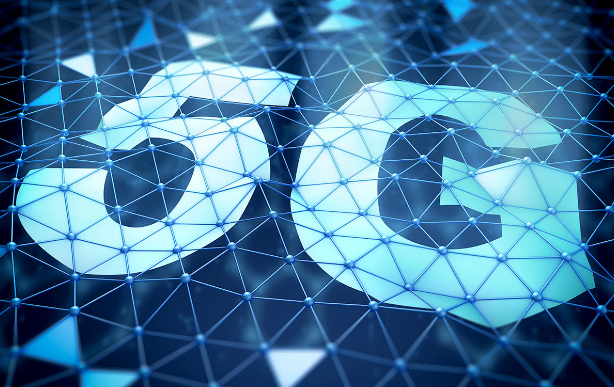
The demand for faster, more reliable mobile communication technologies continues to grow. 5G promises to revolutionize how we connect and interact with the digital world, but do we really understand it? This technology is designed to enhance mobile broadband services, support ultra-reliable low-latency communications, and enable massive machine-type communications. These capabilities are achieved through a combination of advanced technologies and innovative network architectures.
Millimeter Waves
Millimeter waves (mmWaves) are a key enabler of 5G’s high-speed capabilities. Operating in the frequency range of 24 GHz to 100 GHz, mmWaves offer much larger bandwidths compared to the traditional sub-6 GHz bands used in previous generations of mobile networks. This higher frequency spectrum allows for significantly faster data transmission rates, potentially reaching up to 10 Gbps.
However, mmWaves also come with challenges. Due to their high frequency, mmWaves have shorter wavelengths, which leads to limited range and poor penetration through obstacles like buildings and trees. This necessitates the deployment of dense networks of small cells to ensure consistent coverage and performance.
Small Cells
Small cells are low-powered cellular radio access nodes that operate in the range of 10 meters to a few kilometers. They are crucial for addressing the coverage and capacity limitations of mmWaves. Small cells can be classified into three main types:
- Femtocells: Designed for residential or small business use, covering a range of up to 10 meters.
- Picocells: Suitable for enterprises, providing coverage for larger indoor areas like offices and shopping malls.
- Microcells: Used in urban areas to cover distances up to 2 kilometers.
By deploying a dense network of small cells, 5G can achieve high capacity and low latency, ensuring seamless connectivity even in densely populated urban areas. These small cells are typically connected via fiber optic cables or microwave links to the core network, forming an integral part of the 5G infrastructure.
Multiple Input Multiple Output (MIMO)
Another critical technology in the 5G arsenal is Multiple Input Multiple Output (MIMO). MIMO leverages multiple antennas at both the transmitter and receiver ends to enhance communication performance. In 5G, massive MIMO (mMIMO) systems are employed, which can involve hundreds of antennas.
How MIMO Works
MIMO technology exploits the spatial dimension of the wireless communication channel. By using multiple antennas, it can create multiple parallel data streams within the same frequency band. This improves spectral efficiency and increases the data throughput.
There are two main types of MIMO:
- Single-user MIMO (SU-MIMO): Multiple antennas serve a single user, enhancing the signal quality and data rates.
- Multi-user MIMO (MU-MIMO): Multiple antennas serve multiple users simultaneously, improving overall network capacity.
In massive MIMO, the large number of antennas enables beamforming and spatial multiplexing. Beamforming focuses the signal in specific directions, reducing interference and increasing the range. Spatial multiplexing allows multiple data streams to be transmitted concurrently, further boosting the network capacity.
Network Slicing
5G introduces the concept of network slicing, which allows operators to create multiple virtual networks on a shared physical infrastructure. Each slice can be tailored to meet the specific requirements of different applications or services. For example:
- A slice for enhanced mobile broadband (eMBB) can be optimized for high data rates.
- A slice for ultra-reliable low-latency communications (URLLC) can ensure minimal delay and high reliability.
- A slice for massive machine-type communications (mMTC) can support a large number of connected devices with efficient signaling.
Network slicing is made possible by software-defined networking (SDN) and network function virtualization (NFV) technologies, which provide the flexibility and scalability needed to manage diverse service requirements.
Edge Computing
Edge computing is another essential component of the 5G ecosystem. By processing data closer to the source (i.e., at the edge of the network), edge computing reduces latency and improves the performance of time-sensitive applications. This is particularly important for applications like autonomous driving, augmented reality, and industrial automation, where even minor delays can have significant consequences.
Edge computing also helps to offload traffic from the core network, enhancing overall network efficiency and reducing congestion. It involves deploying edge servers and data centers strategically across the network to handle local processing and storage needs.
Spectrum Efficiency and Carrier Aggregation
5G also improves spectrum efficiency through advanced modulation schemes and carrier aggregation. Carrier aggregation allows operators to combine multiple frequency bands to create a wider effective bandwidth, increasing data rates and capacity. This is especially useful in environments with fragmented spectrum allocations.
Moving Forward
5G technology represents a significant leap forward in mobile communications, driven by a combination of advanced technologies and innovative network architectures. Millimeter waves provide the high-speed backbone, while small cells ensure comprehensive coverage and capacity. Massive MIMO enhances spectral efficiency and throughput, and network slicing offers tailored virtual networks for diverse applications. Edge computing reduces latency and improves performance for time-sensitive services.
As 5G continues to evolve, it will pave the way for new and transformative applications, from smart cities and autonomous vehicles to immersive virtual reality experiences. Understanding the technical aspects of 5G technology is crucial for appreciating its potential and the profound impact it will have on our connected world.